Genetic Engineering: Redefining Life’s Blueprin
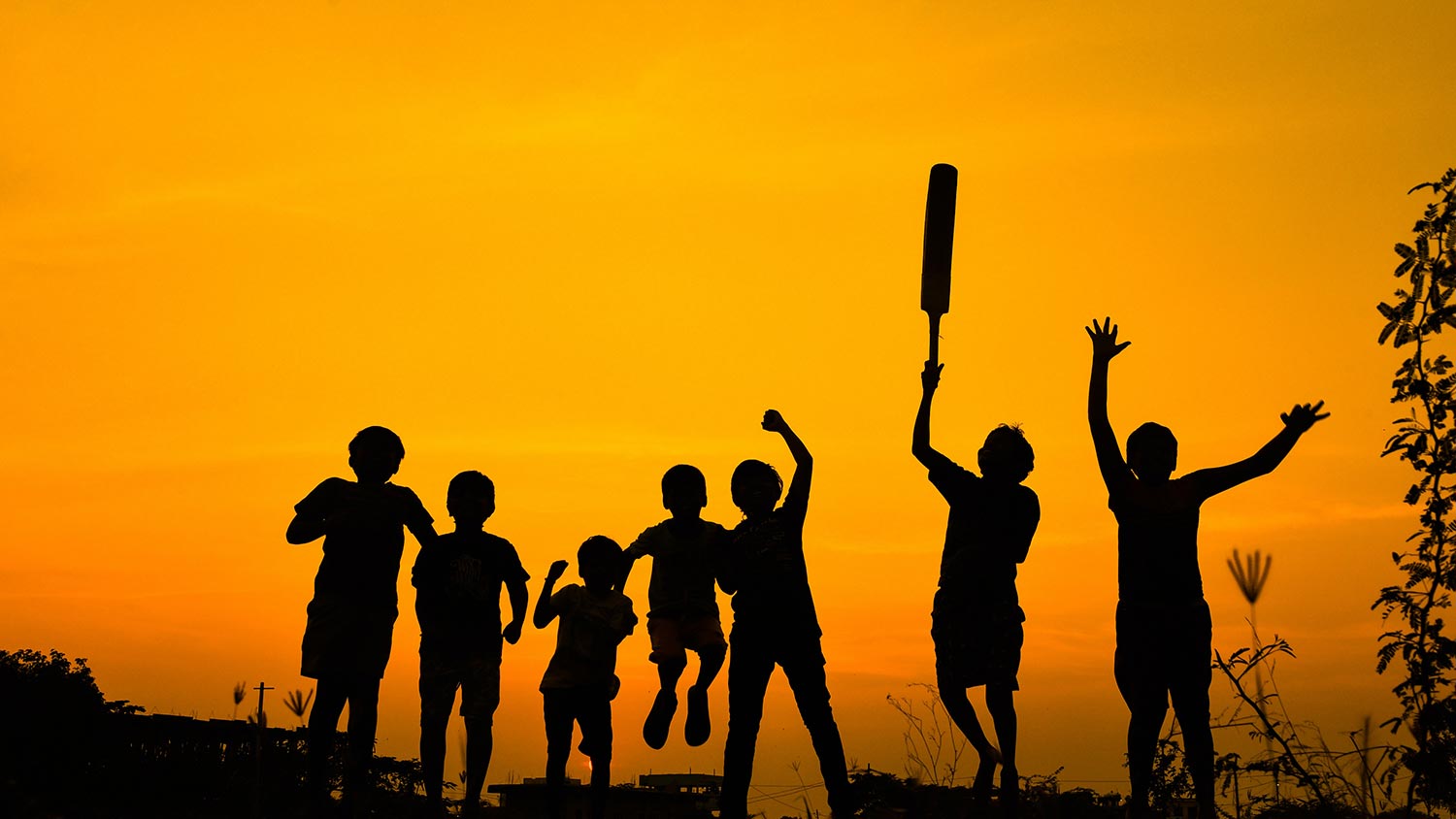
“A gene can be short, consisting of only a few nucleotides, like the genes that code for ribosomes. Ribosomes are organelles that are involved in protein synthesis. Moreover, genes can also be terribly long, made up of millions of nucleotides. For instance, the DMD gene that codes for a protein dystrophin has over 2.2 million nucleotides,” writes Ishana Sharma.
“Genetic engineering” sounds like such a fancy and esoteric term. My first recollection of it is watching Spider-Man and being fascinated by the antagonists like Green Goblin and Dr. Octopus. I remember thinking how terrifying it would be to alter the very fundamentals that make you, well, you. Genetics, as a branch of biology, has never failed to leave me intrigued. Growing up watching movies like Spider-Man and Krrish, reading comics where superheroes can do practically anything and now reading about genetics in biology, I have contemplated how our genetic mechanism is so fundamental yet complex more often than I would like to admit.
I mean, each of Dr. Octopus’s machine-made arms had a mind of their own. They influenced him and swayed his mind, merging the fine line between what he considered right and wrong. Is it not scary to think how inserting a chip in your neck can transform your entire sense of being and instantaneously make you a monster, loved by none but feared by all? Spider-Man himself underwent a plethora of changes after a genetically modified spider bit him. His eyesight improved, and he gained physical strength- something he had only previously dreamt about; his range of auditory sensitivity increased manifold, and he could swing from one building to another within the blink of an eye. Now, I understand that this is all fictional, but the fact that this could be a possibility makes genetics and genetic engineering so interesting.
Genes control everything in our body. How we look, what we like to eat, our height, our hair colour, how we react to our surroundings, how we handle situations and, to a certain extent, our humour and response to challenges are also governed by our genes. In Iceland, a company called deCODE Genetics is where a robot methodically arranges the blood of thousands of people, from which DNA is extracted and placed in sequencing machines. Here, the sequencing of genes is studied, and links are drawn between gene sequences and detailed information about the person, including their diet, habits, personality, hobbies, relationship choices and also the diseases to which they succumbed. Links are established to understand how much of our behaviour has already been determined by our underlying biology. It also enables us to understand what genes certain diseases like Alzheimer’s, cancer and schizophrenia are linked with.
I have used the words ‘genes’ or ‘genetics’ thirteen times so far. So you must be wondering what exactly are genes, and rightfully so. Simply put, genes are sequences of repeating nucleotides that code for specific proteins and are expressed in our body as a feature, either internally or externally. Genetics, moreover, is the study of the transmission of genes, heredity, and variations in inheritance. There are approximately 19,900 genes in a single cell of our body that code for different types of characters in our body. The discovery of DNA, or deoxyribonucleic acid, which is the component of our genes, in the 1860s, carved a path for further development in genetics. In 1973, Herbert Boyer and Stanley Cohen developed genetic engineering by inventing rDNA, or recombinant DNA technology and jumpstarted further advancements in biotechnology. They were the first to insert recombined genes into a bacterial cell so that the foreign DNA could replicate in the cell naturally.
Furthermore, a genetically modified organism (GMO) is one whose genes have been altered to benefit the ecosystem. Insulin was the first consumer product (GMO) developed via genetic engineering that was approved by the FDA to be used on diabetes patients. Lamentably, there would have been a massive diabetes epidemic without insulin, which, like a nuclear fission reaction of Uranium-235, would have led to uncontrolled deaths, and the population demographic would have witnessed a sharp decline. A plethora of regulations and guidelines have also been set that are essential criteria for scientists and biotechnologists to consider before introducing GMOs for public consumption.
The potential for genetic engineering is unparalleled, and its appropriate application is in medicine, agriculture, industrial biotechnology, and research.
Gene editing is a technology that works to alter DNA, i.e., the sequence of nucleotides that make up a gene. A gene can be short, consisting of only a few nucleotides, like the genes that code for ribosomes. Ribosomes are organelles that are involved in protein synthesis. Moreover, genes can also be terribly long, made up of millions of nucleotides. For instance, the DMD gene that codes for a protein dystrophin has over 2.2 million nucleotides. Therefore, through gene editing, scientists aim to either remove or change a single nucleotide or even swap out a whole gene sequence altogether. If you’re thinking about what difference swapping a single nucleotide can make, think again. A single point mutation occurs in an organism when a base pair (abbreviated as A, T, G, C) is changed, removed, or added. People with sickle cell anaemia have just one base that mutates from the A-base to the T-base. CRISPR-Cas9 and TALEN technology are notorious for scintillating a worldwide debate on the ethics of genetic engineering. CRISPR-Cas9 is an enzyme that behaves like DNA ‘scissors’. An RNA strand guides this enzyme to target a specific gene sequence. Depending on what type of gene editing is to be done, this enzyme can remove or add either just a single base pair or introduce a much larger sequence, after which the DNA repairs itself and glues the different parts together. TALEN technology, on the other hand, uses a different approach. It uses a pair of proteins that act like molecular scissors to cut the DNA at a specific location, allowing for the removal or addition of genetic material.
Gene editing is relatively less complicated in single-point mutations, but this exact process is much more extensive for illnesses like schizophrenia and cardiovascular diseases. Using CRISPR, scientists hope to accelerate the process of finding cures for cancer, research on mental health, and also heading towards eradication of diseases like HIV and cancer. Agriculturalists aim to craft drought-resilient crops and effectively deal with climate change before the situation is exacerbated. Next comes Transcription activator-like effector nucleases, or simply TALEN. This was extensively used in the hornless cattle project. The issue lay in the fact that most farm cattle must be dehorned manually, which is a strenuous process for both the animal and the person involved. Dehorning is vital to ensure the safety of other cattle and the handler. It was noted that the genetically modified cattle (GMC) did not undergo drastic physiological changes due to the introduction of new genes via a micro-injection. Although this is at a proof of concept stage, it still goes on to prove how beneficial genetic engineering can be. Gene editing can also be used in vaccine development by genetically modifying bacteria or viruses, enhancing crop resistance to desiccating circumstances, and bioremediation. Genetically modified food involves introducing nutrients or other factors in various vegetables, fruits and meat. This was done in Japan by introducing GABA, a stress-reducing amino acid, in tomatoes. In the 1990s, a company called Calgene innovated the first GMO tomato to improve its longevity, whereas, in 2021, Sanatech Seed added GABA, a neurotransmitter in the brain that produces a calming effect.
However, genetic engineering is a rather controversial facet that raises several questions. Is the technology as accurate as promised? Is taking a risk worth it? Are the resources worth the effort? Are hornless cattle going to help defeat crises like climate change and inequality? Who is going to regulate and set limits, especially for animals? How much is too much? The answer to each of these questions is yet to be ascertained. Scientists are worried that genetic engineering might completely extirpate biodiversity. When it comes to humans, if genetic engineering is made available, future parents will start bringing an endless list of modifications that they want in their child to their doctor. A designer baby is a baby whose genome, or set of genes, has been scrupulously selected, reviewed and altered. This would end neurodiversity entirely and produce a set of clones that may look different on the outside but are precisely the same on the inside. More hazardous than this is the fact that even the tiniest error could have a detrimental impact on the child’s life.
In conclusion, genetic engineering is like the Swiss Army knife of science: versatile, compelling and capable of fixing almost anything. It offers transformative potential across medicine, agriculture, and environmental science, addressing critical challenges like disease treatment, food security, and pollution. Techniques like CRISPR-Cas9 and TALEN have enabled scientists further to improve the efficacy of pharmaceuticals and food products. At the same time, these advancements bring ethical, ecological, and social concerns, widening the socio-economic divide and necessitating scrutinised regulation and public discourse. Genetic engineering still has a long way to go before becoming legal and available for public consumption, with many more hurdles to cross. Whether or not we have taken a step in the right direction is something only time can tell.